May 9, 2022
A Holistic Look At Vertical Farming’s Carbon Footprint And Land Use
Editor's Note: This post was written by Till Weidner, a Postdoctoral Researcher at ETH Zürich with an interest in urban farming and energy. Weidner’s publications include: Energy optimisation of plant factories and greenhouses for different climatic conditions (2021) in Energy Conversion and Management, Regional conditions shape the food–energy–land nexus of low-carbon indoor farming (2022) in Nature Food, and Estimating the potential of building integration and regional synergies to improve the environmental performance of urban vertical farming (2022) in Frontiers in Sustainable Food Systems.
It’s no secret that a host of simultaneous crises have been pressuring the current food system. With a worsening climate, biodiversity loss, a global pandemic, and war, we’re being challenged both from a supply resilience and an environmental sustainability perspective.
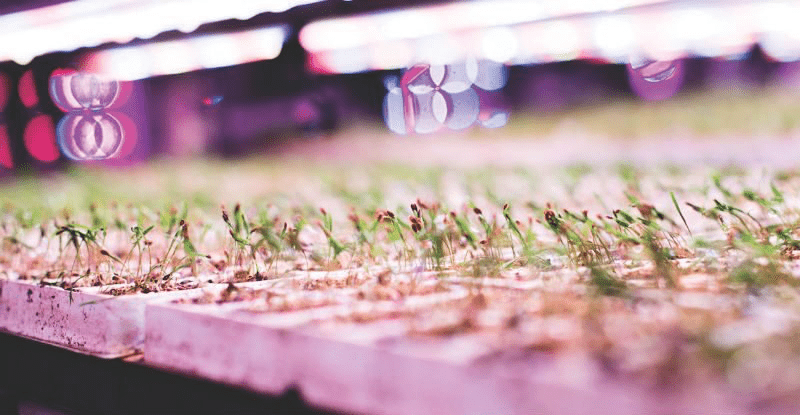
Image sourced from Heliospectra
Vertical farming has slowly become one of the more eye-popping solutions to these growing challenges, attracting billions in private funding.
With all the hype in the industry, many proponents claim that vertical farming is more sustainable than the current food supply, citing reductions in land, water, and pesticide use alongside reduced food miles.
However, environmental sustainability is not a single-issue concept. And, as a result, vertical farms face a lot of criticism about how “sustainable” they truly are. According to respondents of the 2021 Global CEA Census, 70% of CEA operators believe that the industry is susceptible to excessive greenwashing.
We need to consider the holistic sustainability of bringing farming indoors.
Even though there is evidence behind vertical farms significantly reducing freshwater withdrawal and the loss of biosphere integrity from pesticides, the main area of concern is greenhouse gas (GHG) emissions and land use.
Alongside researchers from Michigan State University and the Stockholm Environment Institute, I recently dove into why this is a problem.
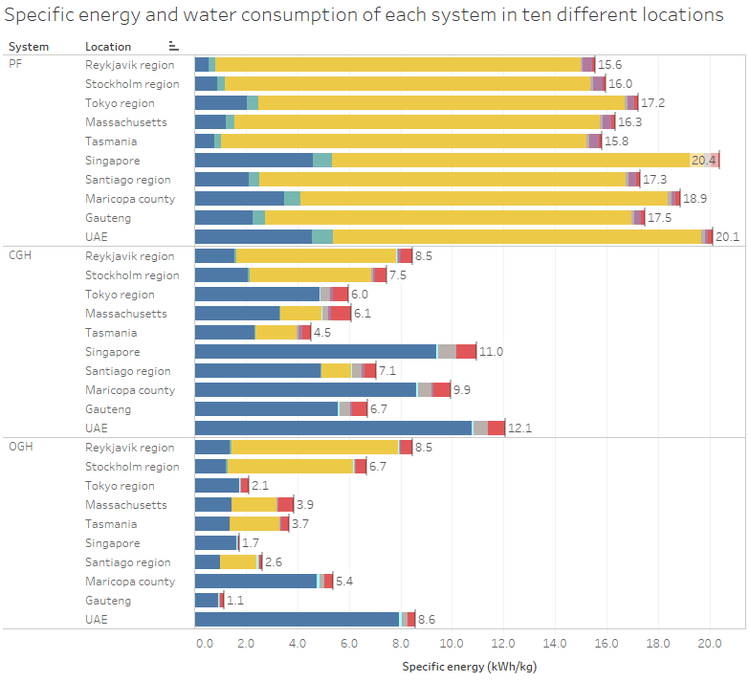
Chart 1: Average annual specific energy consumption of the three growing systems: plant factories (PF), closed greenhouses (CGH), and open ventilated greenhouses (OGH) at different locations. The locations are sorted by their average annual temperature in ascending order. Source: Weidner, T., Yang, A., & Hamm, M. W. (2021). Energy optimisation of plant factories and greenhouses for different climatic conditions. Energy Conversion and Management, 243, 114336. https://doi.org/10.1016/j.enconman.2021.114336
When looking at carbon footprint, we often see claims around the reduction of food miles. In truth, data shows that food miles contribute very little to the total carbon footprint when compared to “on-farm” emissions (this includes energy inputs).
As part of this research, we modeled dynamic CEA systems in 10 different locations (Chart 1), optimizing lighting and climate control to obtain the lowest specific energy consumption (energy spent per unit mass of food produced, in this case a mix of vegetables). This included electrifying air conditioning using heat pumps and refrigeration cycles for dehumidification. Results in Chart 1 indicate that vertical farms require at least 16-20 kWh per kg (depending on the location, main contribution lighting), while open ventilated greenhouses can have much lower specific energy requirements.
Similarly, according to respondents of the 2021 Global CEA Census, indoor vertical farms, on average, use 38.8kWh per kg of harvested crop. Given that the global average carbon intensity of electricity is 475g CO2 per kWh, we can assume that, on average, vertical farms have a carbon footprint of 18.43kg CO2 per kg of harvested crop.
Comparing this to Our World In Data’s calculations on GHG emissions per kilogram of food product - where beef, the highest emitter, emits 99.48 kg CO2, rice emits 4.45 kg CO2, and tomatoes emit 2.09 kg CO2 - vertically farmed produce clearly doesn't live up to its image of sustainability from the perspective of carbon footprint when fossil fuel-sourced energy is used.
While there is potential here for renewable electricity being generated and used in vertical farms, it has also been found that most renewable energy infrastructure (solar photovoltaic and wind turbines) require vast amounts of land, while also having their own unique set of ecological challenges (e.g. the accidental killing of large birds of prey).
Here are the top takeaways on the impacts of renewable energy generation on the total land use of vertical farms.
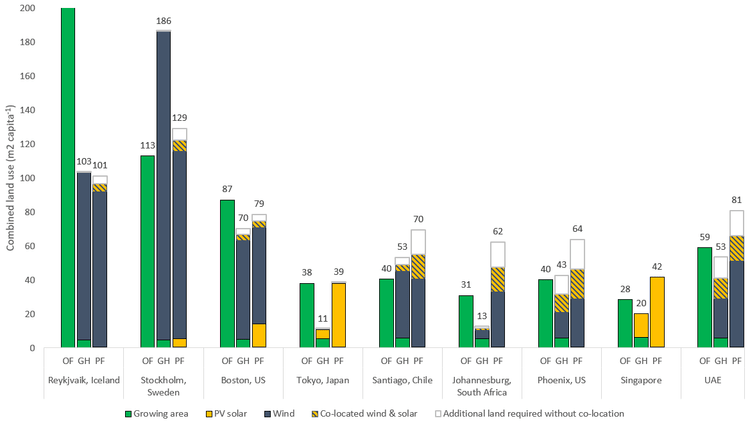
Chart 2: Combined land-use requirements for both growing and renewable energy supply to satisfy annual micronutrient requirements for one person depending on the growing technique (OF = open field, GH = greenhouses, PF = plant factories). The split between wind and solar was economically optimized according to the local availability of renewable energy resources. Source: Weidner, T., Yang, A., Forster, F., & Hamm, M. W. (2022). Regional conditions shape the food–energy–land nexus of low-carbon indoor farming. Nature Food. https://doi.org/10.1038/s43016-022-00461-7
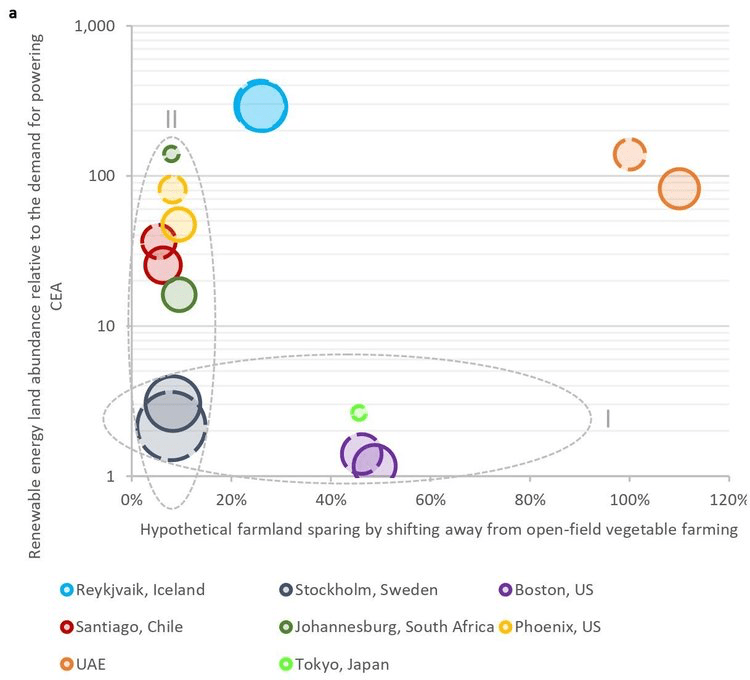
Chart 3: Contextualized regional land-use requirements of greenhouses (dashed-line circles) and plant factories (solid-line circles) to satisfy micronutrient requirements for the local population. Source: Weidner, T., Yang, A., Forster, F., & Hamm, M. W. (2022). Regional conditions shape the food–energy–land nexus of low-carbon indoor farming. Nature Food. https://doi.org/10.1038/s43016-022-00461-7
The direct (growing facility) and indirect (renewable energy generation) land footprint was calculated and compared with open field vegetable farming (Chart 2).
In 8 of 10 locations analyzed, it was found that the indirect land use of vertical farms leads to similar or higher total land footprint than open field farming. Greenhouses were much more favorable in this regard, with greatly reduced total land footprints.
Here, it’s important to note that the picture strongly depends on local conditions; the favorability of one growing method over another can differ from what the combined land footprint indicates.
For example, although combined land use is lower for CEA in Boston, there is limited availability of land for renewable energy generation (cluster 1 on Chart 3). The most favorable locations have vast amounts of land for energy generation and very little suitable farmland (e.g., Reykjavik and the UAE).
It’s clear that while switching to renewable energy greatly reduced the carbon footprint, other impacts such as land use might worsen.
Based on our modeling work, we suggest that to consume energy more efficiently, vertical farms should:
Use energy-efficient LEDs - increased efficiency from 52% to 75% reduces energy requirements by up to 21%.
Moderate the Daily Light Integral (DLI) levels at long photoperiods (the period of daily illumination received by an organism) - up to 20% less energy required when compared to higher DLI levels or shorter photoperiods.
Use water cooling for LEDs - up to 23% reduced energy consumption
Have a climate control system that can simultaneously optimizes for yield and energy - up to 60% reduced energy consumption
Spend more time developing energy efficiency strategies, especially in regards to cooling systems - here, the savings depends on current energy strategies
Note: Indicated energy savings refer to specific energy (kWh per unit mass of produce) of an optimally run facility.
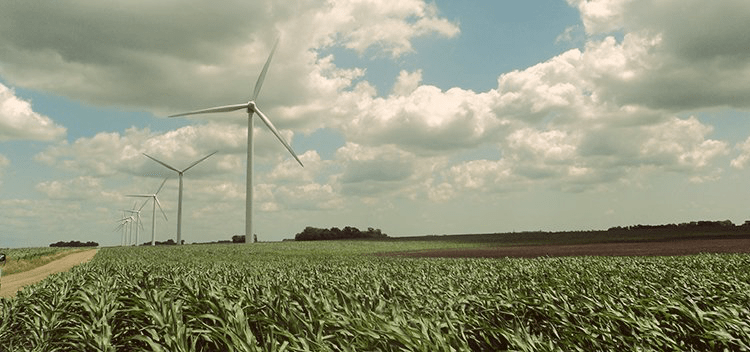
Image sourced from the Clean Grid Alliance
Sourcing energy from renewable sources is also an imperative for sustainability. However, as shown by these publications, it’s important to note that general claims about land savings of indoor farming are often incorrect. The picture strongly depends on the location and the metrics considered (absolute or relative land use).
We have identified a host of further carbon footprint reduction potentials for CEA, including re-using waste heat from air conditioning systems of vertical farms to substitute space heating inputs of nearby buildings, replacing single-use plastic in the supply chain, and sourcing growing medium from waste material. However, they are a drop in the ocean if fossil fuels are used to power a farm. As a result, oftentimes, open-field produce still has a lower carbon footprint.
“For too long, CEA, and especially vertical farming, has been hiding from the difficult discussions on energy, land use, waste, affordability, and carbon footprint. Considering the urgency of the existential challenge humanity faces due to climate change, Agritecture believes that the next generation of CEA game-changing solutions will begin from an honest discussion about sustainability. Data remains more sparse then it should be 10+ years into the vertical farming experiment, but by continuing to open and encourage a data-driven discussion about CEA, Agritecture aims to continue to balance out the hype and greenwashing in the industry. ”
Practitioners should always consider sustainability holistically and avoid greenwashing in their communications. We can only hope to grow this industry sustainably if we can be transparent about the performance of individual vertical farms.